Yb hybrid tweezer-lattice
We are developing a novel experimental platform for quantum simulation with ultracold fermionic Yb atoms. Our main goal is to realize novel techniques for local control in optical lattices by combining optical lattices with optical tweezer arrays.
Quantum simulation of lattice gauge theories
Gauge theories play a fundamental role for our understanding of nature, ranging from high-energy physics to condensed matter. Their formulation on a regularized periodic lattice geometry, so-called lattice gauge theories (LGTs), has proven invaluable for theoretical studies.

However, there exist severe limitations regarding the simulation of real-time dynamics and of systems with finite chemical potential, leaving many important questions unanswered. Recent advances in the controllability of quantum many-body systems have sparked increased interest in quantum simulation of LGTs. In these systems, non-equilibrium phenomena and real-time dynamics are routinely studied over a wide range of parameters. Despite their success, significant progress in simulating LGTs is hindered by the lack of local gauge invariance, which does not naturally occur in these systems. In order to overcome this challenge, we propose to combine ultracold atoms in optical lattices with tweezer arrays, thus uniting global defect-free lattices with precise local control, which in turn allows us to impose local gauge invariance via energy penalties. More specifically, our platform will enable the study of U(1) lattice gauge theories coupled to fermionic matter in one- and two-dimensions. We are further interested in extending these ideas towards quantum simulation of non-Ablian symmetries. One way to do this would be to make use of the SU(N)-symmetric interactions of fermionic Yb atoms.
Recent review:
Cold-atom quantum simulators of gauge theories
Jad C. Halimeh, Monika Aidelsburger, Fabian Grusdt, Philipp Hauke, Bing Yang
preprint arXiv:2310.12201
Ytterbium atoms
Alkaline-earth-(like) atoms such as Yb offer unique opportunities for state-dependent potentials with low scattering rates, high-precision spectroscopy techniques using the ultra-narrow optical clock transition and exotic SU(N) symmetric interactions.
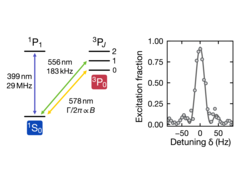
Yb offers several convenient isotopes. We plan to work with the bosonic isotope 174Yb and the two fermionic isotopes 171Yb and 173Yb. While the bosonic isotopes have a nuclear spin I=0, the two fermionic ones have I=1/2 and I=5/2, respectively, which offer unique opportunities for quantum simulation. Yb has two valence electrons, so the internal level structure splits into singlet- and triplet-energy states as shown in the simplified spectrum on the left. The broad transition at 399nm, which connects the states 1S0 to 1P1 is used for slowing the atoms and transverse cooling in a 2D magneto-optical trap. The narrow intercombination line 1S0 - 3P1 at 556nm is used to cool the atoms further in a 3D magneto-optical trap. Since the transition is rather narrow, we use additional slowing beams at 399nm to increase the number of atoms that can be cooled in the green 3D MOT. The excited clock state 3P0 has an extremely long lifetime on the order of 20s, which enables a variety of quantum simulation schemes, where the 3P0 state plays the role of a second species that can experience largely different optical potentials compared to the ground-state 1S0. The two states are connected by an ultra-narrow clock transition at 578nm.
Experimental setup
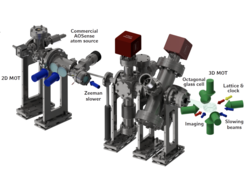
The main source for our experimental setup is a commercial oven from AoSense. It has an integrated Zeeman slower and a 2D magneto-optical trap (MOT). The Zeeman-slowed atoms pass through a differential pumping section and enter the science cell, which is fully made of glass. In the glass cell the atoms are loaded into a 3D MOT that operates at the narrow 1S0 − 3P1 transition. The capture efficiency of the MOT is enhanced using a set of crossed slowing beams on the 1S0 − 1P1 transition. We then load the atoms from the MOT direction into a 3D optical lattice at the clock-magic wavelength 𝜆lat=759.3 nm. We then cool the atoms further using sideband cooling to reach the absolute ground state in each lattice site.
Atom-by-atom assembly in optical lattices
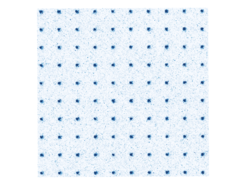
Traditionally, quantum simulation experiments with ultracold atoms start by preparing degenerate quantum gases in optical dipole traps, which are then adiabatically transferred into optical lattices. Typically, this results in rather long cycle times on the order of several tens of seconds. In our experiment we are planning to combine optical tweezer technology with optical lattices to load the atoms directly from the magneto-optical trap into the lattice, thereby reducing the cycle time by at least one order of magnitude. Moreover, the study of real-time dynamics in lattice gauge theories requires the preparation of certain initial states with specific local density distributions, which can be prepared directly with high-fidelity using the assembly technique.
Recent publications:
State-(in)dependent wavelength for neutral 174Yb atoms
As mentioned above, a key ingredient for the quantum simulation experiments planned with Yb atoms is the large variety of state-dependent manipulations that can be realized with rather low scattering rates. However, since the AC polarizabilities for the 1S0−3P0 clock transition are not easy to predict using ab-initio theoretical calculations, measurements of distinctive points greatly aid the accuracy of theoretical models.
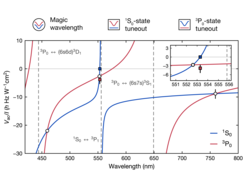
In this work, we performed several measurements to determine the precise location of three distinctive state-(in)dependent wavelengths for 174Yb atoms. Specifically, we determine two magic wavelengths at 652.281(21)THz and 542.50205(19)THz, where the differential light shift on the 1S0−3P0 clock transition vanishes, and one tune-out wavelength at 541.8325(4)THz, where the polarizability of the 1S0 ground state exhibits a zero crossing. The two new magic wavelengths are identified by spectroscopically interrogating cold 174Yb atoms on the clock transition in a one-dimensional optical lattice. The ground-state tune-out wavelength is determined via a parametric heating scheme. With a simple empirical model, we then extrapolate the ground and excited state polarizability over a broad range of wavelengths in the visible spectrum. This model now allows us to predict other wavelengths of interest, such as the location of the 3P0 , i.e., the excited-state tuneout, which will be relevant for realizing our proposal on lattice gauge theories.
Original publication:
State-dependent potentials for the 1S0 and 3P0 clock states of neutral ytterbium atoms,
Tim O. Höhn*, Etienne Staub*, Guillaume Brochier, Nelson Darkwah Oppong, Monika Aidelsburger
* these authors contributed equally to this work
Phys. Rev. A 108, 053325 (2023)
The Team

- Ronen Kroeze, PostDoc
- Tim Höhn, PhD
- Etienne Staub, PhD
- René Villela, PhD
- Leonardo Bezzo, Master student
Former Members
- Dr. Bharath Hebbe Madhusudhana, PostDoc
- Dr. Nelson Darkwah Oppong, PostDoc
- Martí Cladera Rosselló, Master student
- Clara Bachorz, Master student
- Guillaume Brochier, Master student
- Daniel Adler, Master student
- David Gröters, Master student
- Dalila Robledo, Master student
- Semyon Zarutskiy, Intership student
- Tobias Marozsak, Internship student