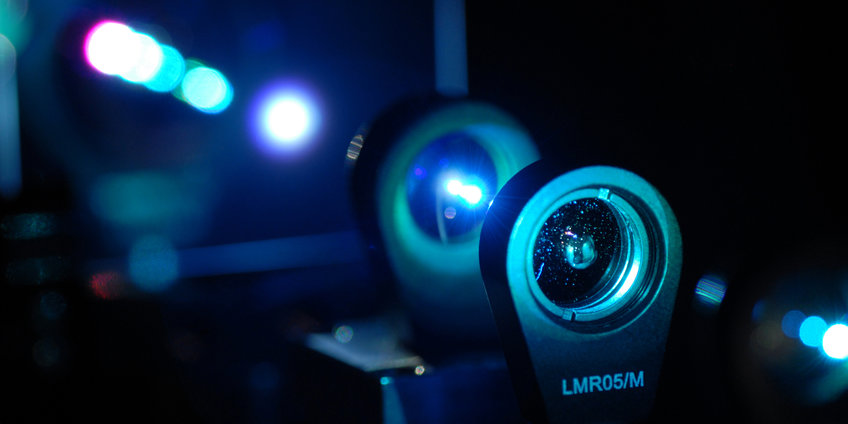
Research at the Max Planck Institute of Quantum Optics
Light can behave as an electromagnetic wave or a shower of particles that have no mass, called photons, depending on the conditions under which it is studied or used. Matter, on the other hand, is composed of particles, but it can actually exhibit wave-like properties, giving rise to many astonishing phenomena in the microcosm.
At our institute we explore the interaction of light and quantum systems, exploiting the two extreme regimes of the wave-particle duality of light and matter. On the one hand we handle light at the single photon level where wave-interference phenomena differ from those of intense light beams. On the other hand, when cooling ensembles of massive particles down to extremely low temperatures we suddenly observe phenomena that go back to their wave-like nature. Furthermore, when dealing with ultrashort and highly intense light pulses comprising trillions of photons we can completely neglect the particle properties of light. We take advantage of the large force that the rapidly oscillating electromagnetic field exerts on electrons to steer their motion within molecules or accelerate them to relativistic energies.
The various scientific topics can be assigned to the following research areas:
Quantum matter at extremely low temperatures
Quantum Many-Body Systems Division, Prof. Immanuel Bloch

At extremely low temperatures (less than one millionth of a Kelvin above absolute zero) systems composed of many quantum particles can take on bizarre properties which go back to their wave properties. For example, an ensemble of a million particles can suddenly behave like one large super atom, with amazing properties like superfluidity and macroscopic interference. These and other exotic properties are of fundamental interest for the understanding of quantum physics. Superimposed by an optical lattice – a crystal lattice of light – quantum gases can serve as versatile model systems for condensed matter physics, helping to understand magnetic and electric properties. Subject to certain conditions, the atoms trapped in the optical lattice could be used for storing quantum information.
Fundamentals of Quantum Optics
Theory Division, Prof. J. Ignacio Cirac
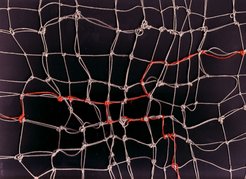
In contrast to classical particles, quantum particles can be found in two states at the same time. For example, quantum bits cannot only take on the values 0 or 1, but all possible combinations of both. Research in the Theory Division centres around the question, how this counter-intuitive behaviour can be exploited in the communication and processing of quantum information. The scientists are exploring new ways of building quantum computers and communication devices which could process and transmit information in an extremely efficient and secure way.
Another focus is the study of complex systems of many quantum particles, the interaction between which can lead to new states of matter. The properties and the behaviour of these systems under the influence of light can be described and characterized with new theoretical tools developed in the Theory Division.
Attosecond and High-Field Physics: Experiments at extremely short time scales
Attosecond Physics Division, Prof. Ferenc Krausz
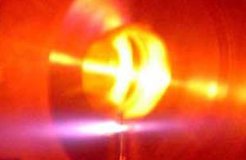
Several attoseconds – billionths of a billionth of a second – is the time it takes for electrons to interact with each other and with light in matter. The inconceivably short period of an attosecond is to a second what a second is to the age of the universe! At MPQ scientists have pioneered the generation and measurement of intense laser light with attosecond control of its electric-field waveform and extreme ultraviolet light flashes of attosecond duration. They use these novel tools for control and real-time observation of the atomic-scale motion of electrons in all forms of matter: inside atoms, molecules, clusters, as well as in solids and plasmas. At ultrahigh laser intensities, MPQ researchers accelerate electrons and ions to velocities approaching the speed of light and pursue the development of compact brilliant particle sources for applications in physics, biology and medicine.
Experiments with single photons and individual atoms
Quantum Dynamics Division, Prof. Gerhard Rempe
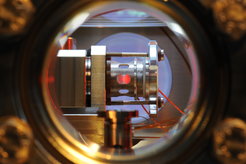
A cubic centimetre of normal air contains about 1020 atoms, a light bulb emits about 1020 photons per second. Scientists at MPQ, however, are able to store and manipulate single atoms and photons and let them interact in a controlled way. Experiments of this kind are an important step towards building a quantum computer, in which quantum particles (such as atoms, molecules or photons) serve as quantum bits. Hybrid quantum systems show particular promise, where single photons transfer quantum information onto individual atoms stored in optical cavities of highest finesse. Subject to certain conditions, atom and photon can form a logical quantum gate, which is the key element in a quantum computer. Several such quantum nodes can be connected to form an extended quantum network.
High-precision spectroscopy of hydrogen and hydrogen-like atoms
Emeritus Group Laser Spectroscopy, Prof. Theodor W. Hänsch

As hydrogen has a very simple atomic structure it is the ideal subject upon which to test the theory of quantum electrodynamics which describes the interaction between light and matter. The development of the frequency comb for which Prof. Theodor W. Hänsch received the Nobel Prize in Physics 2005 led to a great improvement in the measurement of the spectral lines of hydrogen. With the aim of further testing fundamental laws of physics, the division of Prof. Hänsch is extending such investigations to anti-hydrogen, muonic hydrogen, and other elements that resemble hydrogen. These measurements reveal information about universal constants like the fine-structure constant or the charge radius of the proton.
At present, the frequency comb is used in a number of applications, for instance in the calibration of spectrographs at large, ground based telescopes, or in the “dual comb” spectroscopy of complex molecules.