2-for-1 Accelerator
Researchers at the Laboratory for Attosecond Physics in Garching have built the first-ever laser-driven particle accelerator that can generate pairs of electron beams with different energies
Particle accelerator-based radiation sources are an indispensable tool in modern physics and medicine. Some of the larger specimens, such as the LHC in Geneva or the European XFEL in Hamburg, are among the most complex and costly scientific instruments ever constructed. Now, laser physicists at the Laboratory for Attosecond Physics (LAP), which is run jointly by the Max Planck Institute of Quantum Optics (MPQ) and Munich‘s Ludwig Maximilians University (LMU), have developed a laser-driven particle accelerator that is not only capable of producing paired electron beams with different energies, but is also much more compact and economical than conventional designs.
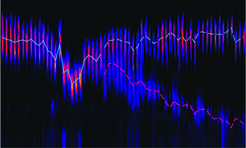
If high-energy radiation sources are ever to become standard tools in research laboratories and radiology departments, ways must be found to make them smaller and much less expensive. Stefan Karsch and his group at the Laboratory for Attosecond Physics, run by LMU and MPQ in Garching, are making steady progress towards this goal. As laser physicists, they are constantly in search of ever more efficient light-driven methods for the acceleration of subatomic particles. The professor's work builds on the chirped pulse amplification technique developed by Donna Strickland and Gérard Mourou to increase the intensity of ultrashort laser pulses (for which they won the Nobel Prize in Physics in 2018).
High-power laser pulses are at the heart of a particle-accelerator concept known as laser wakefield acceleration. When such a pulse is focused onto a gas jet, its wave front first detaches electrons from the gas molecules to form a plasma, and its oscillating electric field creates a plasma wave on which some electrons can surf and gain energy. Together, these effects can rapidly accelerate electron bunches to extremely high speeds over very short distances. Since the electric fields transported by the plasma wave are a thousand times more powerful than those attainable in conventional accelerators, a compact laser system can be used to accelerate electrons to velocities of up to 99.9999 percent of the speed of light within a distance of a few millimetres. These high-energy electron bunches can be used to investigate the ultrafast dynamics characteristic of the subatomic realm or to generate high-intensity X-radiation for medical use.
However, there is a problem with this approach: As a consequence of the extreme conditions in such a plasma accelerator, the plasma waves are prone to instabilities, which are difficult to control. Now three members of the Karsch team – Johannes Wenz, Andreas Döpp and Konstantin Khrennikov – have simultaneously implemented two methods of controlling the trapping process of electrons in the wakefield. Their measurements demonstrate that this makes it possible to produce twin electron bunches with individually tunable energies.
This feat not only represents a significant breakthrough in the control of laser-driven particle accelerators, it opens new perspectives for research on the behaviour of matter on ultrashort timescales. The results lay the foundation for a new generation of experiments in ultrafast dynamics for the new method generates paired electron bunches that are only a few femtoseconds apart (a femtosecond is one millionth of a billionth of a second). These electrons, or the synchrotron radiation associated with them, can therefore be used for pump-probe experiments on the rapid vibrational motions of molecules or other fast-paced aspects of atomic behaviour. So far, such experiments have been restricted to a few compatible combinations of pump and probe sources. The advent of the new technique will provide bursts of electrons and/or multiple terahertz to gamma-ray region photon pulses for this purpose, which are also synchronized to the primary high-power laser pulse.
The Karsch group has already embarked on the construction of the next generation of their novel radiation source. With the ATLAS-3000 laser in LMU‘s new Center for Advanced Laser Applications (CALA), they are commissioning one of the most powerful lasers in the world. Potential medical applications of the newly acquired ability to create dual-energy electron bunches can now be explored, such as the development of compact, laser-driven X-ray sources for diagnostic purposes. (AD/TN)