Digital holography with multiple colours
With the help of special light sources, the three-dimensional shape and spectrum of objects can be recorded completely without photographic lenses. The trick behind is a highly sophisticated form of interference in space and time.
At the end of the 1940s, the Hungarian physicist Dennis Gàbor was working on improving the resolution of his electron microscope. He was wondering about how to use the complete light information for imaging. Gábor wanted to record and reproduce not only the brightness of the light, but also the shape of the wave fronts. In this way, he created the basis of holography: the generation of three-dimensional images of objects.

Lasers are the key to holograms
In practice, Gàbor's experiments had initially only moderate success, because the light sources available were unsuitable for his experiment. It was only after the invention of the laser in 1962 that it became possible to record holograms from which three-dimensional images could be recreated. These images seem to float in space and, when viewed from different directions, actually allow different perspectives on an object. For his pioneering work on holography, Gàbor was awarded the Nobel Prize in Physics in 1971.

A hologram is a two-dimensional superposition pattern that contains all the information about the light field from which it is generated. It entails not only the intensity, but also the phase of the light wave: Does a wave crest or a wave trough reach the photo plate, or something in between? To record such interference patterns, one usually uses coherent light – that is, light in which the wave crests and troughs are in a fixed relationship to each other. Laser light fulfils this requirement.
To record a hologram, the laser light is split into two beams: One illuminates the object, the other serves as a reference for light unaffected by the object. When they are superimposed, a pattern is created that contains information about the obstacle on which one beam was scattered. In conventional holography, this is recorded on a fine-grained photographic film. If the interference image is later illuminated again with the easily produced reference light, the original information can be elicited from it. The light waves then continue to travel as if the object was standing in the space behind the hologram. This creates the 3D images familiar from the security elements on identity cards or credit cards: here, the interference pattern is imprinted on a foil. When viewed in the light, a three-dimensional image appears.
Today, digital holography is becoming more and more popular for special applications. Here, the interference patterns are not recorded on film but with a digital camera sensor or calculated directly by a computer. For the reconstruction, there are spatial light modulators, similar to tiny computer screens, which are used, for example, for near-eye displays in augmented reality applications. Unfortunately, neither camera sensors nor digital displays have enough pixels yet to compete with traditional film in the visual representation of three-dimensional images.
Spatial images are not always the goal
For many applications, it is more interesting to evaluate the digital data of a holographic image in the computer in such a way that the three-dimensional structure of the objects can be calculated from the interference patterns. In a way, the computer takes over the task of the reference light source: as a mathematical transformation. The 3D data obtained in this way can then be used to do everything that computers can do well with 3D objects: Views from all directions and distances.
However, the advantage of lasers to emit light in only one wavelength limits two important aspects of holograms: They do not contain colour information. Plus, the information about the third dimension – the depth – is not unambiguous: you cannot tell from the wave crest whether it was created exactly at the assumed location or one or more entire wavelengths away.
The colourful laser comb
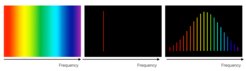
A novel method that can eliminate both limitations is based on a very special light source: frequency combs, an invention by Theodor Hänsch, Director at the Max Planck Institute of Quantum Optics (MPQ), for which he was awarded the Nobel Prize in Physics in 2005.
Frequency combs owe their name to the appearance of their spectrum: an everyday lamp, for example, shines in an entire wavelength range. Laser light, on the other hand, appears as a single, sharp line of a specific wavelength. Frequency combs contain many such laser-like lines at regular, defined intervals – like the tines of a comb. To generate such light, one uses a laser pulsed for a very short time. The duration and sequence of the laser pulses determine the appearance of the comb: the shorter the pulses, the wider the wavelength range covered by the comb. The slower the pulse sequence, the more tines are created.
Beats like on the guitar
With such frequency combs, the frequencies of other laser sources can be measured in a tricky way: By superimposing another light wave on a frequency comb, one obtains an increase and decrease in amplitude, a beat. Anyone who has ever tuned a guitar knows this as a clearly audible acoustic rise and fall. It occurs when two strings produce almost, but not exactly, the same tone. The frequency of the beat corresponds to the difference between the two frequencies involved. In this way, high frequencies can be reduced to lower ones which are thus measurable.
This superposition even works with two frequency combs: A short-pulse laser emits this special light with regular frequency intervals. It then hits a second frequency comb in which the prongs are slightly closer together or further apart, that means is pulsed slightly differently. This difference gives you a beat for each prong of the combs – an interference pattern that changes over time. And that is full of information.
"We are now combining the superposition of light from two frequency combs with holography," summarises Nathalie Picqué, the inventor of the method. Picqué and her colleagues developed this method and first described it in the journal Nature Photonics in November 2021. In May 2022, Nathalie Picqué and her colleague on the project, Edoardo Vicentini, were also awarded the Helmholtz Prize for Metrology for this invention.
Four dimensions: Space and wavelength
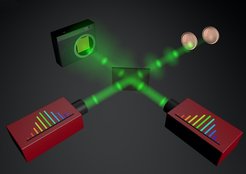
By superimposing the light of two differently pulsed frequency combs, spatial interference patterns are created for all tines of the frequency comb. This would also happen if instead of the frequency combs, for example, the light of a red and a green laser were used simultaneously for illumination. However, the resulting temporal interference would be immeasurably fast. Unlike the hologram with this normal laser light, the interference patterns of the only slightly different comb frequencies vary relatively slowly in time: they flicker with frequencies in the range of a few oscillations per second. This is the optical equivalent of the beating of the barely out-of-tune guitar sides. "From the flickering interference patterns, we calculate a stack of holograms in different wavelengths," Picqué explains. "This gives us the process of 4D holography with the three spatial dimensions and the spectral information as the fourth dimension."
The MPQ researchers demonstrated their method on two coins whose 3D images they created using the new multicolour holography. Unlike with classical holography, they did not have to live with the ambiguity of spatial depth at intervals of wavelength. Because this ambiguity occurs at a different length for each tine of the frequency combs, a much larger range of distances can be unambiguously determined mathematically. Picqué: "With this method, small and large objects can now be measured without contact with a spatial accuracy of nanometres." Such a measurement method is very relevant for materials testing and the life sciences.
Dual comb hyperspectral digital holography 1080p
Applications near by
The fourth dimension that can be reconstructed from the data set is the spectrum of light. And this also offers the potential for interesting applications: If the light path is influenced by a gas, the gas molecules remove part of the spectrum. The team showed that this works in practice by introducing ammonia into the light path. And indeed: "Exactly at the expected wavelength, the intensity of our spectrum decreases," the researcher is pleased to say. "So, we were able to show that our method can also be used to analyse gas samples."

Another important feature of the method is that it does not require imaging optics. This means that the images are not affected by blurring effects due to the distance to the lens. The effect of all focal lengths is determined in a single image. In contrast to classical light microscopes, for example, each image plane can later be cut out of the data on the computer and viewed on the screen in full sharpness and with full colour information – and all this with an exposure that takes only a few seconds.
Where is the journey with dual-comb holography heading? "With cameras of higher spatial and temporal resolution - in other words, more pixels and faster recordings – we can further increase the quality," says Nathalie Picqué, looking to the future. The technology could be miniaturized to such an extent that it fits on an A4 sheet of paper.